The research activity of the group is focused on the identification of new strategies to improve skeletal muscle regeneration. In more detail, the team is studying the role of electrical activity and local trophic factors in the microenvironment of adult skeletal muscle fibres and of satellite cell niche in the post-mitotic myogenesis.
In more detail, the molecular mechanisms controlling the adaptive mechanisms of neuromuscular communication and the regenerative capacity of the skeletal muscle progenitors are investigated. Isolated skeletal muscle fibres are used as cell model to study the plasticity of the neuromuscular junction and skeletal muscle progenitors (satellite cells), murine cell line and immortalized human myoblasts are studied in vitro to identify, in a controlled environment, the pathways regulating the regenerative potential of the skeletal muscle. Particular attention is posed on the contribute of the mechanotransduction to myogenic proliferation and differentiation. The experimental planning includes the use of electrical field stimulation, electrophysiological recordings, biochemical techniques, recombinant synthesis of matrix proteins, immunofluorescence and videoimaging.
The final aim is to discover new strategies to counteract the impaired functionality of the neuromuscular system and the related skeletal muscle atrophy due to ageing and diseases.
Currently, three are the main research projects.
1) Neuromuscular junction plasticity
Expression, distribution and stability of nicotinic acetylcholine receptor (nAChR) at the endplate are controlled by both release of soluble factors from motor nerve endings and electrical activity of muscle fibres. Skeletal muscle denervation causes nAChR cluster dispersal at the endplate zone, reduction in the half-life of synaptic nAChRs, isoform switch from adult (e-nAChRs) to embryonic (g-nAChRs), the latter being diffusely distributed over the muscle plasma membrane.
The effects of the electrical activity are mediated by an increase in intracellular Ca2+ concentration ([Ca2+]i). Evidence is accruing in favour of an inositol 1,4,5-trisphosphate receptor (IP3R)-mediated postsynaptic Ca2+ signalling through which skeletal muscle fibres decode the motoneuron stimulation pattern into local Ca2+ signals involved in the stabilization of the endplate. A functional interplay between nAChRs activity and IP3R1-driven Ca2+ release has been proposed. Interestingly, in pathophysiological conditions, abnormal activity of IP3Rs could be responsible for Ca2+ overload observed in the slow-channel myasthenic syndrome and for NMJ degeneration during excessive cholinergic activation.
Although hypotheses have been formulated for the presence and role of subsynaptic IP3R-driven Ca2+ signalling in innervated skeletal muscle fibers, it is not known whether and how innervation controls the subsynaptic IP3R1 membrane compartment.
With this research activity, the group intends to investigate the molecular mechanisms controlling distribution and function of the subsynaptic IP3R-sensitive Ca2+ store and the the effects of related subsynaptic Ca2+ signals on the metabolic stability of the nAChRs.
The results of the proposed research will unveil important aspects of the neuromuscular junction plasticity not only in pathological conditions but also in extreme environments (i.e., microgravity conditions). In this light, they will be useful for identification of novel strategies to treat neuromuscular diseases associated with altered nAChR stability on ground and to counteract the negative effect of the microgravity on the endplate, which represent the first step leading to the severe muscle atrophy in astronauts during space flights. For this reason the research project received financial support by Italian Space Agency (Grant NEMUCO DC-VUM-2016-068 and NEMUSY DC-DSR-UVS-2022-212).
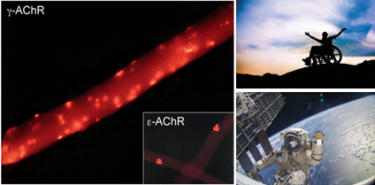
Distribution of embryonic (g-nAChRs) and adult (e-nAChRs) nAChR isoforms. The nAChRs distribution is severely affected by denervation and microgravity.
2) Electrical stimulation of cultured skeletal muscle cells as an in vitro model of exercise
The activation of skeletal muscle fibres by spinal motor neurons can be mimicked by means of electrostimulation techniques used in rehabilitation procedures to counteract age-related neuromuscular disabilities, microgravity-induced atrophy during space flight to degeneration in paraplegic patients. In conditions where exercise is not possible, electrical stimulation mimics beneficial effects by stimulating satellite cells, increasing skeletal muscle fibre size and modulating mitochondrial function. Studying the molecular mechanisms by which exercise influences the functional potential of muscle and other tissues, including the nervous system, is of great relevance.
We use in-vitro exercise models based on appropriate field electrostimulations of cultured skeletal muscle cells mimicking the moderate or intense contractions and the related values of reactive oxygen species (ROS) production analysing the under-studied effects of specific antioxidants usable for human health.
We also study how electrical activity participates in the autocrine and paracrine regulation of the regenerative capacity of skeletal muscle stem cells. In particular, we analyse the production of local trophic factors and myokines which, acting as hormones, influence the activity of other organs such as bone and brain.
Thanks to new-generation fully programmable electrical stimulators, it has been possible in our laboratories to use “noisy” waveforms obtained from recordings of human muscle electrical activity. They were more effective than the stereotyped pulses generally used in the clinic, because varying randomly the amplitude, duration and frequency, they reduce pain and muscle fatigue during the electrical stimulation. We are now studying the relationship between the stimulation pattern and the ammount of miokines released hoping to optimise the electrostimulation protocols today used in clinic in order to improve their efficiency.
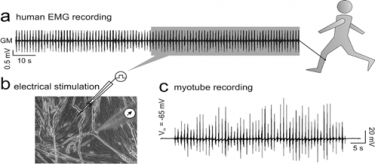
A trace sampled from a human EMG recording to design the “noise” stimulation pattern (a) delivered to cultured myotubes (b). The magnificazion of the “noisy” stimulus reveals the stochastic nature of the burst activity (c).
3) Piezo1 and mechanotransduction in skeletal muscle regeneration
Since the discovery of the two mechanically-activated ion channels Piezo1 and Piezo2, the comprehension of the mechanotransduction mechanisms has made great strides. Piezo1 is a cationic transmembrane channel able to “sense” and convert mechanical stimuli into physiological cell signals. Piezo1 is activated by variations of the plasmamembrane tension due to the phospholipidic composition (force from lipid) and by the membrane interactions with the extracellular matrix and the cytoskeleton (force from filament). By doing this, Piezo1 controls proliferation, migration and differentiation of different type of cells. Moreover, the chemical activation thanks to the identification of specific antagonist, has made Piezo1 a powerful pharmacological target for the prevention and the treatment of many pathologies.
Our lab has been one of the first that has identified Piezo1 in myogenic precursors and adult skeletal muscle fibers. We hypothesize that Piezo1 could be an important transductor of mechanical signals muscle cells, such those generated during contractions. Our aim is now the characterization of the transduction pathways controlled by Piezo1, particularly those involved in the control of membrane excitability and intracellular calcium signalling. The outcome of our study is the development of new powerful strategies having Piezo1 as novel pharmacological tool to counteract the effects of aging, disuse and injuries on skeletal muscle functionality.
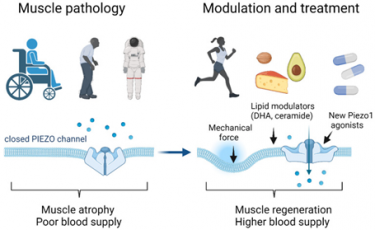
Potentiation of Piezo 1 activity by physical exercise, diet and chemical/pharmacological activation can counter the effect of muscle disuse, aging and unloading (from Bernareggi et al., Int J Mol Sci. 2022 doi: 10.3390/ijms23126616).