Our research aims to identify the general principles governing assembly and remodeling of synaptic connections in the mammalian brain. Understanding the molecular mechanisms that control development and function of neural networks is essential for treating neurological and psychiatric disorders.
Quite possibly the most complex organ in the body, the brain determines our behaviors, thoughts and identity. Activity-dependent changes in brain structure and function enables us to form new memories and learn new skills. Alterations in brain connectivity and excitability can however lead to disabling brain disorders, such as epilepsy, autism, depression and ataxia.
Brain function: Focus on cell adhesion molecules and calcium channels
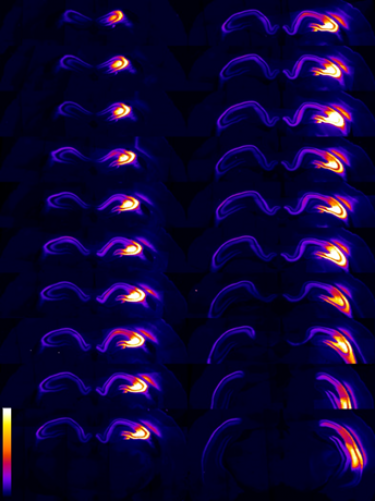
Brain function critically depends on how neurons interact and communicate with each other at synapses. Our goal is to identify the molecular mechanisms governing formation, specialization and remodeling of synaptic connections in the mammalian cortex.
Synapses can be viewed as both learning and memory storage devices. On the one hand, they are highly ‘plastic’, changing how they transmit information in response to changes in brain activity. On the other hand, they can be highly ‘tenacious’, with many synapses retaining their structural and functional properties over many years. Our overarching interest is in how synapses achieve the right balance between plasticity and tenacity, and how disrupting this equilibrium contributes to neurological disorders.
In particular, we investigate how (i) synaptic cell adhesion molecules determine synapse specificity and (ii) how alternative splicing of calcium channels contributes to synapse diversity. We approach these questions from molecular, network and behavioral perspectives, to gain a comprehensive understanding of brain function.
We are committed to using our discoveries to develop effective treatments for brain disorders to improve patients’ health and quality of life.
Current projects
Synaptic magneto-genetics
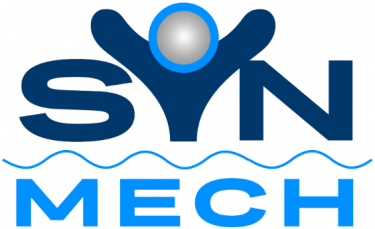
www.synmech.eu
Our team is dedicated to developing an innovative technology called synaptic magnetogenetics that will allow for the precise control of neural circuit connectivity in the brain. We believe that this technology has the potential to revolutionize the way we treat high-prevalence treatment-resistant brain disorders.
Magnetogenetics is an emerging field of health science that merges the strengths of optogenetics and magneto-mechanical stimulations. Like optogenetics, magnetogenetics employs actuators to target specific neural circuits, while exploiting magnetic fields, rather than light, to achieve non-invasive brain stimulation.
Here, we implement an innovative solution for magnetogenetics based on biocompatible magnetic nanoparticles and bioengineered synaptic mechanosensors that work together to regulate brain connectivity in response to focused magnetic fields, delivered via high-permeability transcranial magnetic stimulators. We assess the capacity of the synaptic mechanogenetic toolkit to normalize network activity in mouse models of stroke, epilepsy, autism and depression.
Collaborators: University of Verona, Forschungszentrum Julich (Germany), Brain Science tools (The Netherlands), CNR (Milan), Deutsches Zentrum für Neurodegenerative Erkrankungen (Germany), Universitair Medisch Centrum Utrecht (The Netherlands) and Ca’ Granda Hospital (Milan).
Project supported by the European Research Council (SynMech; Horizon-EIC-2022-Pathfinderopen-01-01; grant ID: 101099579), the Cariplo Foundation (DIGAP; grant ID: 2019-3438) and the Ministry of Health (DRACULA; Finalized research; grant ID: GR-2021-12375009).
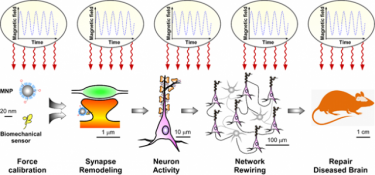
CRISPR/Cas9-dependent regulation of alternative splicing as an all-purpose genetic therapy for episodic ataxia and absence epilepsy
We have developed a CRISPR/Cas9-mediated genome editing strategy to control alternative splicing as an all-purpose genetic therapy for neurological disorders caused by loss-of-function mutations in the synaptic calcium channel CaV2.1. These disorders include episodic ataxia type 2 and absence epilepsy.
Optogenetic experiments show that our approach is highly effective in rescuing network excitability defects in human iPSC-induced neurons carrying CaV2.1 mutations, as well as electrophysiological and behavioral abnormalities in CaV2.1-deficient mice.
Collaborators: Pediatric Hospital Gaslini (Genoa).
Project supported by the Telethon foundation (grant ID: GGP19181), the Telethon and Cariplo foundations (grant ID: GJC22053) and the University of Trieste.
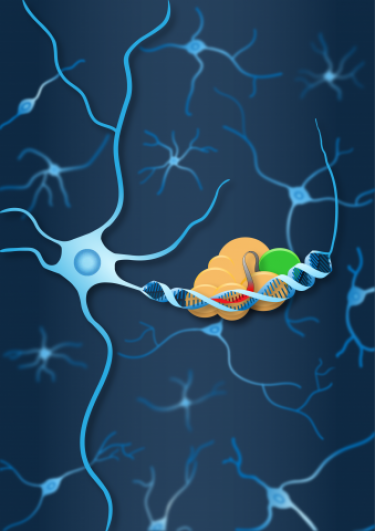